On the Trail of Important Life Processes
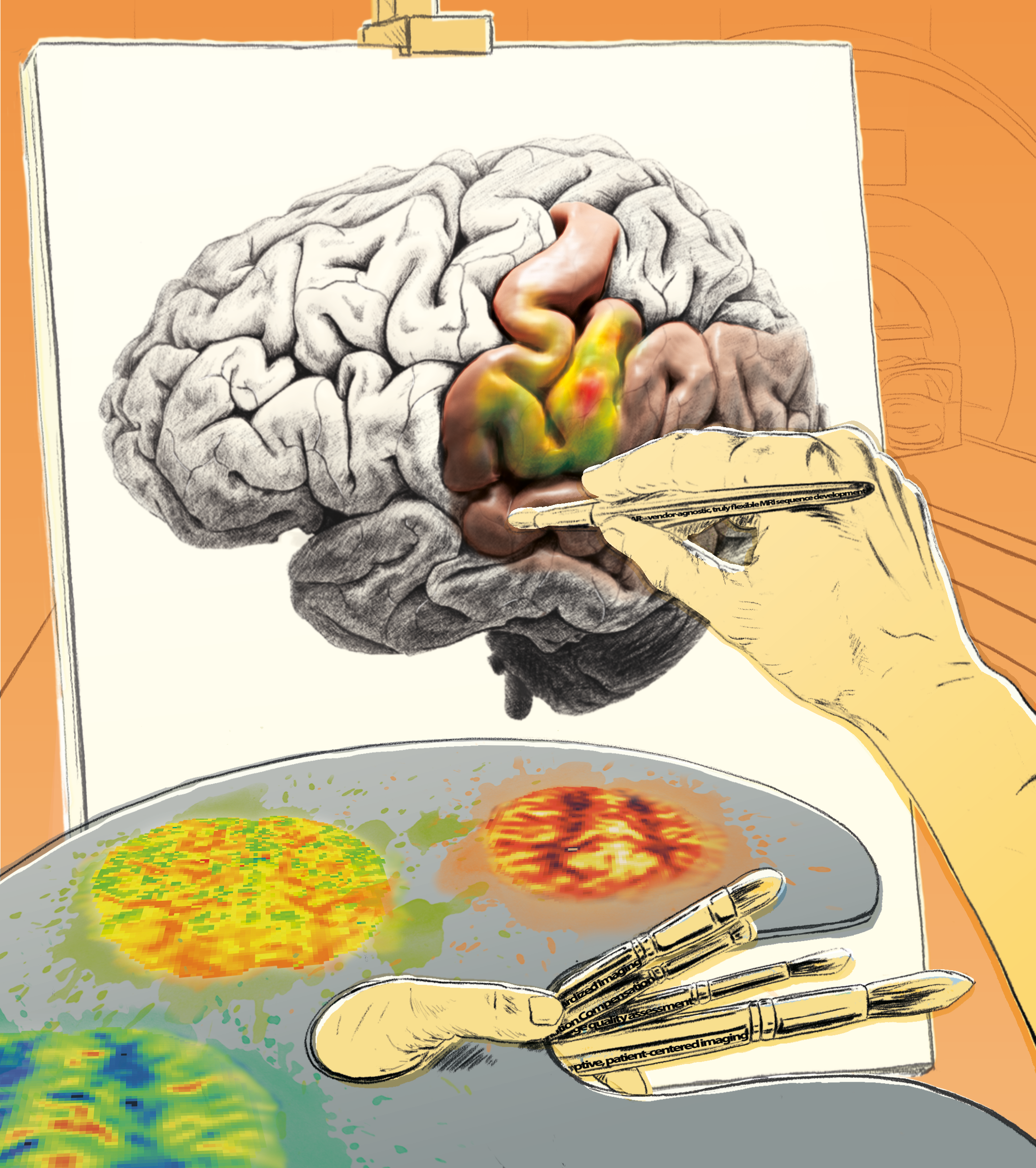
Ultrasound and MRI are among the most important imaging procedures in medicine. The Fraunhofer Institute for Digital Medicine is developing software solutions that optimize image acquisition and simplify tracking physiological functions.
An MRI scanner can create non-invasive 3D images from inside the body. Generally, the device acquires still images. However, this technology can do much more: it can make vital processes visible, such as the flow of blood into the finest of brain vessels. “Physiological imaging can sometimes help detect diseases earlier than a conventional MRI scan,” says MEVIS researcher Daniel Hoinkiss. “For example, a stroke initially manifests itself as a disruption in the brain’s blood supply and only later as a structural change in a conventional MRI image.”
Physiological imaging could also uncover both neurological diseases such as Alzheimer’s and Parkinson’s as well as tumors at an early stage. The earlier the diagnosis, the better the likelihood that a treatment succeeds. Physiological MR imaging has, however, only been employed in the clinical routines of a few hospitals due to the difficulty of operation and the susceptibility to errors. Physiological imaging is much more complex than a conventional MRI scan. Prior to each examination, specialized personnel are required to set various parameters and adapt them to each individual patient.
Fraunhofer MEVIS wants to overcome this hurdle. The goal is to make physiological imaging as easy to acquire as standard MR imaging to increase accessibility for routine diagnostics. “The personnel only need to press a button, and the software automatically adapts the MR sequence to the patient,” explains Hoinkiss’ colleague Nora-Josefin Breutigam. Describing their vision, she says, “Afterwards, all that remains is to wait until the image is acquired.”
An algorithm that compensates for movement
A combination of several innovative methods should facilitate this. One method should solve the following problem: Image acquisition inside the scanner can take up to several minutes. Most patients inevitably move during this time, which unsurprisingly interferes with image acquisition. “We want to counteract this with an adaptive technique that continuously compensates for this movement,” explains Hoinkiss. “If the software recognizes, for example, that the patient slipped one millimeter to the side, it corrects the MRI scanner so that it acquires the same part of the body as before.”
The experts pay particular attention to MRI blood flow measurement, which does not rely on using a contrast agent. In this method, blood in the neck is marked magnetically. After this blood reaches the brain, an MRI scan is initiated. Comparing this with a reference measurement allows visualization of blood flow into the brain in the time period between marking and recording. The timing of the MR images plays a major role in this technique; it is important to seize the exact moment when the marked blood is distributed in the brain. “This happens differently for each person," explains Breutigam. “This is why we are developing software that ensures that the MRI scanner doesn't select the wrong moment.”
The researchers are also developing an algorithm that automatically suppresses irrelevant signals. Observing physiological processes requires identifying extremely small changes in the signal. Therefore, it is essential to suppress types of tissue that aren’t involved. The new algorithm is designed to do exactly this, thereby emphasizing the relevant signals even more clearly.
Fast ultrasound
Physiological imaging is also gaining increasingly significance in another procedure: ultrasound. A physician performing an ultrasound needs special training and a lot of experience to operate the ultrasound equipment optimally. Fraunhofer MEVIS is planning systems to ease operation of this technology, particularly for young specialists. “Software will analyze the ultrasound images in real time and determine, for example, whether the image contains errors,” explains MEVIS physicist Sven Rothlübbers. “This should then be shown to the operator.”
The researchers at Fraunhofer are also refining ultrasound technology itself. They are developing a method to measure the temperature of tissue during image acquisition. This is valuable for cancer treatments in which the tumor is destroyed by applying heat. A further field of research is 3D ultrasound image acquisition. Vast amounts of data have to be handled here, resulting in fairly lengthy recording times. “We are working on self-learning procedures that generate high-quality images based on only a few input data,” says Rothlübbers. “This could significantly accelerate the image acquisition process.”
Algorithms like these could also be beneficial when using ultrasound with contrast agents. During image acquisition, a contrast agent is administered, which travels through blood vessels and appears on the image with high contrast. “The speed at which it becomes light and dark again says a lot about what is happening,” explains Sven Rothlübbers. “This could, for example, make tumors and cysts in organs such as the liver or kidney more visible and distinguishable.”